Hydrogels, a class of hydrophilic polymers capable of holding large amounts of water, have garnered significant attention due to their versatile applications, particularly in biomedical fields. These materials can be categorized into synthetic and natural hydrogels, each possessing unique characteristics that can be tailored through various modification strategies. This article delves into the advanced modification techniques of hydrogels and their implications, particularly highlighting the expertise of Alfa Chemistry in enhancing hydrogel performance for specific applications.
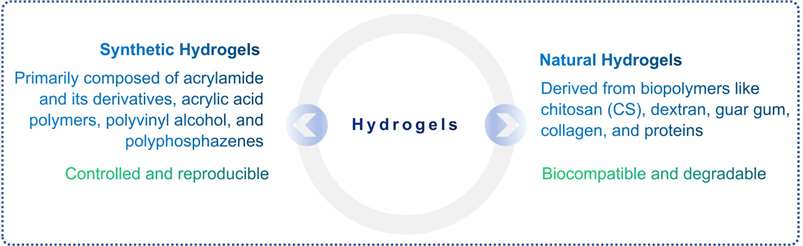
Alfa Chemistry Hydrogel Series Products
View a wide range of graphene-based aerogel products to find the best option for your application:
Modification Techniques for Enhanced Hydrogel Performance
Chemical Grafting and Crosslinking
Chemical grafting involves the attachment of functional groups or polymers to the hydrogel backbone, improving its mechanical properties, stability, and responsiveness. For instance, PVA hydrogels can be grafted with succinic anhydride to introduce carboxyl groups, enhancing their interaction with biological tissues. Chemical crosslinking methods also enable the formation of stable three-dimensional networks, crucial for applications that require sustained mechanical integrity.
Crosslinking modifications in protein-based hydrogels utilize the inherent reactive side chains, such as amino, hydroxyl, thiol, phenol, and carboxyl groups, to form new polymer structures. Crosslinking agents, like polycarboxylic acids, enhance the water absorbency and stability of protein hydrogels, making them suitable for biomedical applications like wound dressings and artificial organs.
Physical Blending and Composite Formation
Physical blending combines hydrogels with other polymers or nanoparticles to form composite materials with superior properties. For example, blending PVA with gelatin-a collagen derivative with excellent biocompatibility-creates a composite hydrogel that retains gelatin's favorable properties while overcoming its inherent brittleness and solubility issues. This technique is widely employed by Alfa Chemistry to create hydrogels with tailored mechanical and thermal properties for specific biomedical uses.
Composite formation with inorganic fillers, such as calcium phosphate or bioactive glass, further enhances the hydrogel's mechanical strength and bioactivity. Similarly, organic molecules like hyaluronic acid, collagen, and chitosan are used to create hybrid hydrogels that offer improved structural and functional characteristics for tissue engineering and regenerative medicine.
Smart Hydrogel Modifications: Enhancing Responsiveness
Smart hydrogels are engineered to respond to external stimuli, such as pH, temperature, or ionic strength, making them ideal for applications requiring controlled drug release or responsive biomaterials. Modification techniques include creating macroporous or superporous hydrogels, interpenetrating polymer networks (IPNs), and nanocomposite hydrogels, all of which improve the responsiveness and functionality of the material.
Physical crosslinking is a key approach in smart hydrogel modification. It involves forming hydrogels under specific environmental conditions, such as changes in temperature or pH, resulting in a network that can undergo reversible sol-gel transitions. These hydrogels, like PEG-PLA and PEG-PBT block copolymers, are particularly valuable in the biomedical field due to their low toxicity and ease of degradation.
Fig.1 Various external stimuli, including pH, temperature, electricity, magnetics, light, and biomolecules (including glucose and enzyme), are controlling the drug release from a smart hydrogel[1].
Novel Modification Approaches in Hydrogel Engineering
Nano-Hydrogel Synthesis Methods
The synthesis of nanogels, a subclass of hydrogels with particle sizes ranging from 20 to 300 nm, utilizes several advanced techniques to achieve precise control over particle size and distribution:
Emulsion Polymerization | This method involves the formation of nanogel particles through the polymerization of monomers dispersed as tiny droplets in a water phase containing surfactants. Nanogels synthesized via this method, such as PEO-PPO-PEI copolymers, are used for encapsulating hydrophobic drugs, enhancing their solubility and bioavailability. |
Radiation Polymerization | Employing γ-rays or electron beams to induce polymerization, this technique produces nanogels with high purity and uniform crosslinking. This approach can allow the development of biocompatible nanogels for sensitive biomedical applications such as drug delivery and diagnostics. |
Self-Assembly Techniques | Self-assembly involves the spontaneous organization of molecules into well-defined structures through non-covalent interactions. This method is particularly effective for creating multifunctional nanogels, capable of targeted drug delivery, prolonged circulation, and stimuli-responsive release. |
Interpenetrating Polymer Networks (IPNs) | IPNs are formed by the entanglement of two or more polymer networks, offering enhanced mechanical stability and tunable responsiveness. By crosslinking each polymer network independently, IPNs achieve superior strength, elasticity, and durability. This approach is widely used in designing hydrogels with complex architectures, such as those incorporating silica or other nanomaterials to form porous structures capable of precise drug delivery and biosensing functions. |
Fig.1 Formation and structure of semi- and fully interpenetrating polymer networks (IPNs)[2].
Biomedical Applications of Modified Hydrogels
- Wound Dressings and Artificial Organs
High-absorbency hydrogels, also known as superabsorbent polymers, have been successfully employed as wound dressings due to their ability to absorb large volumes of exudates without adhering to the wound site. PVA-based hydrogels are also used in artificial tissues, such as synthetic muscles and cartilage, offering excellent biocompatibility and moisture retention properties.
- Tissue Engineering and Cell Cultivation
Modified PEG hydrogels, crosslinked with bioactive molecules like vascular endothelial growth factor (VEGF) and transforming growth factor (TGF-β1), serve as scaffolds that enhance cell adhesion, proliferation, and extracellular matrix production. These properties make them suitable for tissue engineering applications, such as cartilage, skin, and liver regeneration.
- Drug Delivery Systems and Microcapsules

Hydrogels modified with responsive functionalities are widely used in drug delivery systems, where they provide controlled release profiles tailored to specific therapeutic needs. The encapsulation of drugs within hydrogel microcapsules helps protect sensitive molecules, such as insulin, from degradation, while the hydrogel matrix provides a sustained release mechanism, reducing dosing frequency and enhancing patient compliance.
- Biosensors and Smart Materials
Smart hydrogels that respond to pH, temperature, or other external stimuli are increasingly used in biosensors for detecting biomolecules like antigens, enzymes, and proteins. These hydrogels enable rapid, sensitive, and specific detection, making them invaluable in diagnostic applications.
Conclusion
The modification of hydrogels is a rapidly evolving field with profound implications for biotechnology, medicine, and materials science. Alfa Chemistry continues to lead the way in hydrogel innovation, driving forward new possibilities in drug delivery, tissue engineering, and smart materials through meticulous research and development.
References
- Bordbar-Khiabani A., et al. (2022). "Smart Hydrogels for Advanced Drug Delivery Systems." Int. J. Mol. Sci., 23(7), 3665.
- Bayat M., et al. (2019). "Injectable Microgel-hydrogel Composites "Plum pudding gels": New System for Prolonged Drug Delivery." Nanomaterials for Drug Delivery and Therapy, 343-372.
Please kindly note that our products and services are for research use only.